Understanding Quantum Computing
Quantum computing represents a significant shift in how we approach problem-solving and computations, differing fundamentally from traditional binary computing. At the core of quantum computing lies the concept of the qubit, or quantum bit. While classical computers use bits that are either 0 or 1, qubits can exist in a state of superposition, allowing them to be both 0 and 1 simultaneously. This characteristic immensely increases the potential for parallel processing, enabling quantum computers to solve complex problems at speeds unachievable by conventional means.
Another critical concept in quantum computing is entanglement. When qubits become entangled, the state of one qubit will instantly influence the state of another, regardless of the distance separating them. This phenomenon allows for vastly enhanced communication and computation capabilities. The implications of both superposition and entanglement are profound, as they offer a pathway to achieve computing power that far exceeds that of today’s most advanced supercomputers.
To further elucidate, consider the example of a quantum computer attempting to factor large integers, a task that currently poses a challenge for traditional computers. The ability to leverage superposition means that a quantum machine can examine multiple potential factors simultaneously, drastically reducing the time required for calculations. While this capability is still in its infancy, companies and research institutions are diligently working to harness the power of quantum computing for a variety of applications, including cryptography, optimization problems, and complex simulations.
In summary, by understanding these foundational principles—qubits, superposition, and entanglement—readers can appreciate the remarkable potential of quantum computing. The advancement of this technology holds promise to revolutionize industries and fundamentally alter our computational capabilities in the near future.
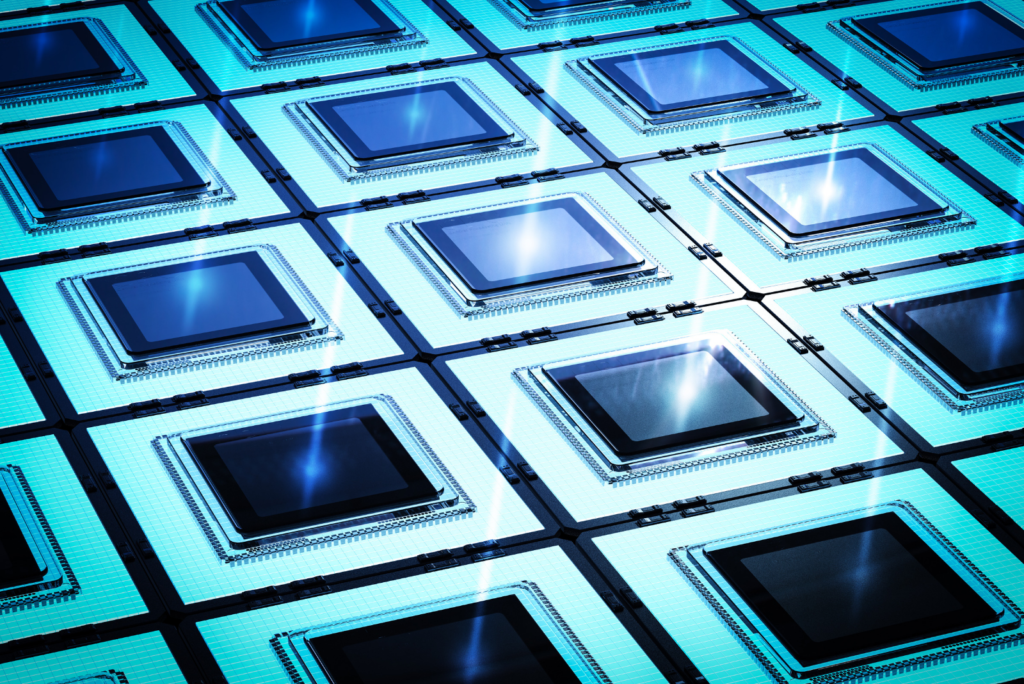
Current Applications and Innovations
Quantum computing is rapidly emerging as a transformative force across multiple sectors, given its capability to handle complex computation exponentially faster than classical computers. One of the most prominent applications is in the field of cryptography. Quantum computers are being developed to break traditional encryption methods, which may leave current security paradigms vulnerable. For instance, companies like IBM are exploring quantum algorithms capable of cracking RSA encryption systems, thereby pushing organizations to rethink data security measures.
In the realm of medicine, quantum computing is utilized for drug discovery and personalized medicine. By simulating molecular interactions at an unprecedented scale and speed, researchers can identify potential drug candidates more efficiently. A notable example is the collaboration between D-Wave and pharmaceutical companies that focuses on optimizing drug formulations through quantum capabilities, expediting the arrival of effective treatments for diseases.
The financial sector also stands to gain significantly from the advantages of quantum computing. Quantum algorithms are being employed for risk analysis, portfolio optimization, and fraud detection. For instance, a recent case study demonstrated how a hedge fund utilized quantum computing to analyze vast datasets, resulting in improved predictive analytics and better investment strategies.
Additionally, material science is experiencing a paradigm shift due to quantum computing. Innovations in simulating material properties, such as superconductors and novel alloys, enable researchers to design materials with desirable attributes more swiftly. The partnership between Google and researchers from various universities is a prime example, where quantum simulations are being used to develop high-temperature superconductors.
Overall, the current innovations in quantum computing are touching on critical domains like cryptography, medicine, finance, and material science, demonstrating how this technology is shaping the future and enhancing operational efficiencies across various industries.
Challenges in Quantum Computing Development
Quantum computing represents a groundbreaking shift in computational capabilities; however, the journey to realizing its full potential is fraught with challenges. One significant technical hurdle is qubit coherence. Qubits are the fundamental units of information in quantum computers, yet they are notoriously fragile. Their ability to maintain quantum states is often short-lived due to interactions with their environment, which can lead to decoherence. This greatly impacts the reliability of quantum computations and presents a key obstacle in developing stable, long-lasting qubits that can perform complex calculations reliably.
Another critical issue is error rates. Quantum systems are inherently susceptible to errors caused by a variety of factors, including thermal fluctuations and electromagnetic radiation. These errors can disrupt quantum operations, leading to incorrect outputs. To mitigate this, researchers are exploring various quantum error correction codes. However, implementing effective error correction significantly increases the complexity of quantum systems, thereby posing additional logistical challenges.
Beyond technical issues, scalability also presents a significant challenge in the realm of quantum computing. Current quantum processors typically feature a limited number of qubits, constraining the complexity of problems that can be solved. As researchers aim to scale quantum systems, the integration with classical computing infrastructure becomes crucial. Compatibility with existing technologies is essential for transitioning quantum computing from theoretical frameworks to practical applications.
Despite these challenges, advancements in materials science and quantum algorithms hold promise for overcoming existing barriers. Innovative approaches like topological quantum computing and developments in superconducting qubits are being actively researched. Addressing these multifaceted issues will be pivotal in refining quantum computing technologies, ultimately paving the way for their widespread adoption in various fields, including cryptography, optimization, and complex modeling.
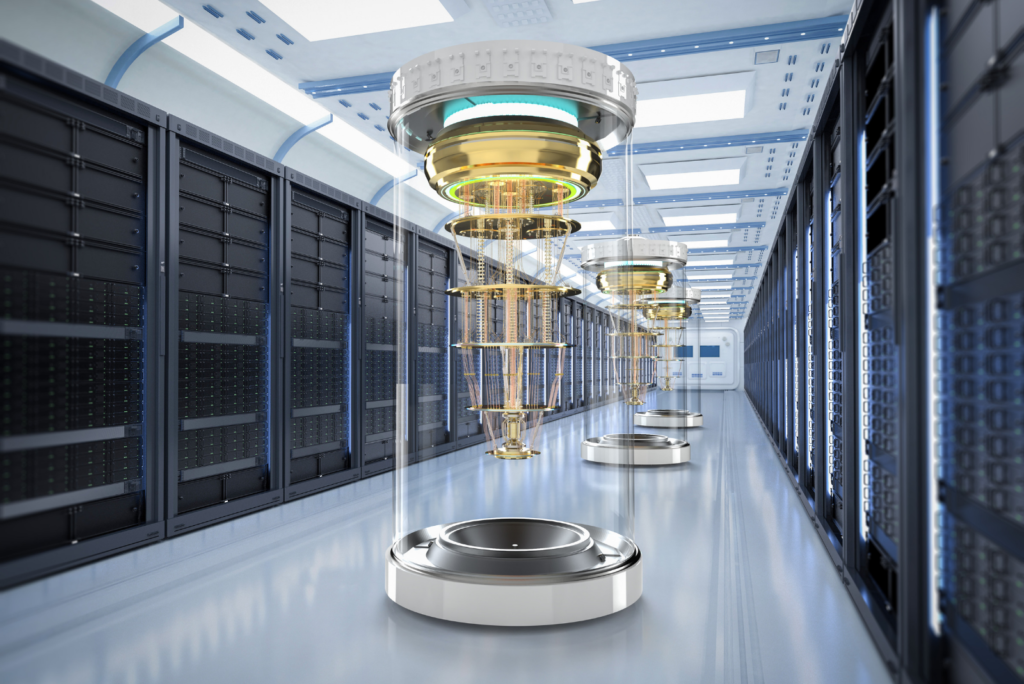
The Future of Quantum Computing
As we progress further into the 21st century, the future of quantum computing presents a compelling landscape ripe with potential advancements that could significantly transform various aspects of society. Quantum computing, characterized by its ability to perform calculations at unprecedented speeds using quantum bits or qubits, promises to solve complex problems that classical computers struggle with. Predictions suggest that in the coming years, we may witness a drive toward achieving practical quantum supremacy, wherein quantum computers surpass traditional systems in specific tasks.
Governments and private sectors around the world are taking proactive steps to foster innovation in quantum technologies. Collaborations between public institutions and private companies are becoming more prevalent, with financial investments aimed at research and development. Countries like the United States, China, and members of the European Union are laying out strategic plans to enhance their quantum research capabilities, acknowledging the competitive edge that superiority in this field could provide. This collaborative environment is likely to catalyze rapid advancements, driving breakthroughs that can be utilized in various industries, from cryptography to drug discovery.
As quantum computing becomes more integrated into our daily lives, its impact on consumers is expected to expand. From improved online security solutions derived from quantum cryptography to AI advancements that may result from enhanced computational power, the ripple effects will be vast. However, alongside these promising prospects, ethical considerations arise. Concerns about data privacy and the potential for misuse of quantum technologies are paramount. The responsibility will lie with developers, policymakers, and society at large to establish frameworks ensuring that quantum advancements are approached with caution, balancing innovation with ethical obligations. These discussions will be essential as we embrace the unprecedented capabilities that arise from quantum computation.